2018
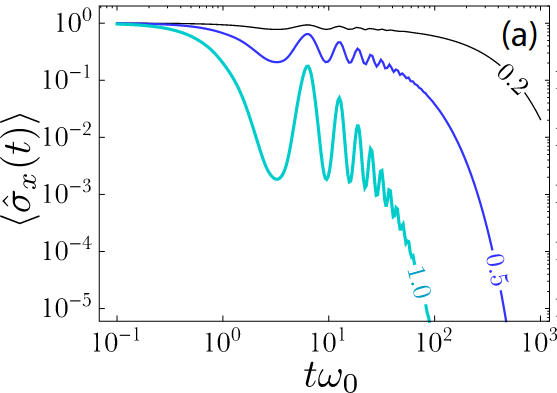
Analog quantum simulation of extremely sub-Ohmic spin-boson models – M. Abdi and M. B. Plenio, Physical Review A, 98, 040303 (2018)
DOI: doi.org/10.1103/PhysRevA.98.040303
2D materials with an embedded colour center, that is an optically active electron spin, offer novel ways to control the motional dynamics of such membranes. Here we are exploring the potential of this set-up for simulating the dynamics of the eponymous spin-boson model in which a spin interacts with an environment composed of a large number of bosonic degrees of freedom. The action of the environment is characterised by its spectral density, essentially a product of mode density and coupling strength. When the spectral density is growing slower than linear, this is referred to as the sub-Ohmic spectral density which is particularly challenging to simulate classically. This motivated us to explore whether this model can be realised under realistic experimental conditions. This works answers this question in the positive.
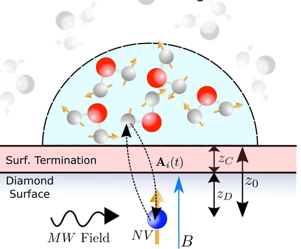
Sensing phases of water via nitrogen-vacancy centres in diamond – P. Fernández-Acebal and M. B. Plenio, Scientific Reports, 8, (2018) 13453
DOI: doi.org/10.1038/s41598-018-31745-3
*This work is licensed under Creative Commons Attribution 4
Undoubtedly, water is one of the key substances for life being present in many biological systems. Still, our current understanding of water, more concisely, of its properties at the molecular level, is rather small. Exotic phases of water at low temperatures have been predicted and detected but even if a complete understanding of water dynamics at a nanometric scale is highly desirable, it proposes an onerous challenge for commonly-used detectors.
In this paper, we propose the nitrogen-vacancy (NV) center in diamond as a high-resolution sensor that assists in the investigation of exotic phases of water. The NV center possesses an electronic spin that is extremely sensitive to changes in the magnetic field within its surroundings. By an adequate control of the NV center, we can make its spin sensitive to the fields emanating from the protons conforming the water molecules. The sensitivity of the NV center is such that structural changes of water, such as phase transitions, will be perceived via some modifications on the magnetic field sensed by the NV center, making out of it an ideal phase transitions sensor at the nanoscale and thus, opening the route to the investigation of exotic phases of mater.
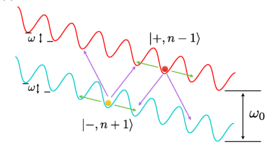
Observation of Floquet Raman Transition in a Driven Solid-State Spin System – Z. Shu, Y. Liu, Q. Cao, P. Yang, S. Zhang, M. B. Plenio, F. Jelezko, and J. Cai, Physical Review Letters, 121, 210501 (2018)
DOI: doi.org/10.1103/PhysRevLett.121.210501
Periodically driven quantum systems can display temporal structures that are in many ways reminiscent of spatial structures of matter. For example, time translation invariance may be broken and a Floquet time crystal is formed.
In this paper we examine such Floquet physics experimentally in an NV center and demonstrate that control of such dynamics is possible.
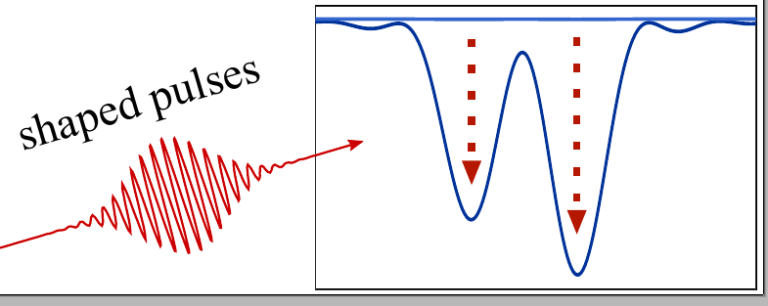
Shaped Pulses for Energy-Efficient High-Field NMR at the Nanoscale – J. Casanova, Z.-Y. Wang, I. Schwartz, and M. B. Plenio, Physical Review Applied, 10, 044072 (2018)
DOI: doi.org/10.1103/PhysRevApplied.10.044072
The nitrogen-vacancy (NV) center in diamond is a minute detector opening the door for nanoscale nuclear magnetic resonance (NMR). To sense the signal, one applies a sequence of microwave pulses on the NV center to modulate its electron spin dynamics so that the NV center is sensitive to NMR signals instead of environmental noise. Conducting the sensing experiment at a high static magnetic field has several advantages, such as large spin decay times and a large chemical shift which is a key quantity in molecular structure determination. However, it is very challenging to perform the nanoscale NMR at a high static magnetic field, because standard methods requires an exceedingly large microwave power.
In this work, we address these challenges with the design of shaped pulses. We present energy efficient control schemes for high field nuclear magnetic resonance detection at the nanoscale. The low intensities of the applied microwave control fields also facilitate applications in biological settings where heating effects need to be avoided as they can damage samples and affect biological function. The method is general and can be applied to any quantum sensor subjected to pulsed control sequences.
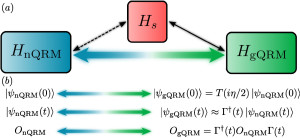
Connecting nth order generalised quantum Rabi models: Emergence of nonlinear spin-boson coupling via spin rotations - J. Casanova, R. Puebla, H. Moya-Cessa, and M. B. Plenio, npj Quantum Information 4, 47 (2018)
DOI: doi.org/10.1038/s41534-018-0096-9
*This work is licensed under Creative Commons Attribution 4
The interaction between a spin-1/2 particle and a bosonic mode is one of the most fundamental models in quantum mechanics. In particular, when the interaction between the subsystems comprises a linear exchange of quantum excitations, that is, when the spin state changes by absorbing or emitting only one bosonic excitation, the model is known as quantum Rabi model. This celebrated mode is of paramount importance not only for a comprehensive description of light-matter interaction, but also because it describes different physical systems such as trapped ions or circuit QED, it has thus become a milestone in the development of quantum-based technologies and quantum information processing.
There are yet other possible interaction mechanisms between a spin-1/2 particle and a bosonic mode beyond the linear case. Indeed, it may be possible that the spin state changes at the expense of emitting or absorbing n>1 bosonic excitations. Such a nonlinear interaction dramatically modifies the system’s properties with respect to its linear counterpart. These nonlinear models are however typically hard to control or even to implement. In this work we have shown that, despite these nonlinear models fundamentally differ from the linear one, their physics can be well approximated by a model comprising a linear interaction plus simple spin rotations. Indeed, among different applications, our theory indicates that a linear quantum Rabi model allows for the simulation of nonlinear interacting systems without the actual need of implementing the nonlinear spin-boson couplings.
This unexpected connection between this family of interacting systems may open new avenues in the quantum simulation of nonlinear systems with potential applications in quantum-based technologies.
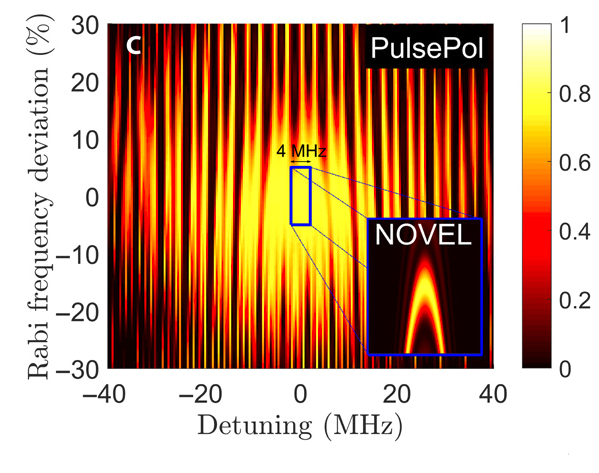
Robust optical polarization of nuclear spin baths using Hamiltonian engineering of nitrogen-vacancy center quantum dynamics – I. Schwartz, J.Scheuer, B. Tratzmiller, S. Müller, Q. Chen , I. Dhand, Z. Wang, C. Müller, B. Naydenov, F. Jelezko and M. B. Plenio, Science Advances, 4(8), eaat8978 (2018)
DOI: 10.1126/sciadv.aat8978
*This work is licensed under Creative Commons Attribution-NonCommercial license
Dynamic nuclear polarization (DNP) is an important technique that uses polarization transfer from electron to nuclear spins to achieve nuclear hyperpolarization. As nitrogen-vacancy (NV) centers can be optically polarized to almost unity polarization, which if transferred to nuclear spins can increase their polarization >10,000-fold. Combining efficient DNP with NV centers offers promising opportunities for novel technological applications, including nanoscale nuclear magnetic resonance spectroscopy of liquids, hyperpolarized nanodiamonds as magnetic resonance imaging contrast agents, and the initialization of nuclear spin–based diamond quantum simulators. However, none of the current realizations of polarization transfer are simultaneously robust and sufficiently efficient, making the realization of the applications extremely challenging. We introduce the concept of systematically designing polarization sequences by Hamiltonian engineering, resulting in polarization sequences that are robust and fast.
In this work we theoretically derive sequences and experimentally demonstrate that they are capable of efficient polarization transfer from optically polarized NV centers in diamond to the surrounding 13C nuclear spin bath even in the presence of control errors. This paves the way for making the abovementioned novel applications possible.
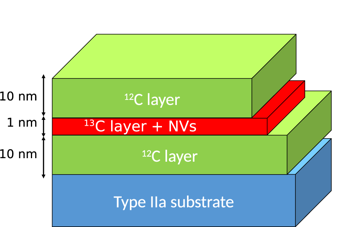
Coherent control of solid state nuclear spin nano-ensembles – T. Unden, N. Tomek, T. Weggler , F. Frank , P.London, J. Zopes , C. Degen , N. Raatz, J. Meijer, H. Watanabe, K. M. Itoh , M. B. Plenio, B. Naydenov and F. Jelezko, npj Quantum Information, 4, 39 (2018)
DOI: https://doi.org/10.1038/s41534-018-0089-8
*This work is licensed under Creative Commons Attribution 4
Quantum simulators are devices that can emulate the dynamics of other physical systems in a highly controlled fashion, providing access to dynamics that cannot be simulated efficiently on a classical computer. Currently the most successful implementations of such quantum simulators are using trapped ions cooled to micro-Kelvin temperatures or atoms in optical lattices cooled to nano-Klevin regime where both are held in ultrahigh vacuum and are shielded from external influences. In 2013 we suggested a radical departure from this approach by using 2-dimensional layers of nuclear spins chemically bonded to a diamond and initialised, controlled and read-out by a close-by NV center to provide an architecture for a quantum simulator that can operate at room temperature and ambient conditions. In the present work we are taking some steps towards the realisation of this design with the construction of an ultrathin 13C layer inside of diamond and the exploration of the spin polarisation and control of this layer by means of nearby NV-centers.
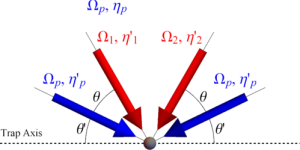
Double-path dark-state laser cooling in a three-level system – J. Cerrillo, A. Retzker, and M. B. Plenio, Physical Review A, 98, 013423 (2018) | ArXiv
DOI: doi.org/10.1103/PhysRevA.98.013423
*The figure was reprinted with the permission from the above paper. Copyright 2018 American Physical Society
Sideband cooling is an important technique that enables ground state cooling of trapped ions and other physical systems. It relies on the fact that a red detuned laser field does not have quite enough energy to excite the ion so that the systems has to borrow a phonon from its vibrational mode to make up for the energy difference. In a tight trap, the subsequent spontaneous decay will typically not generate a phonon. The principle in use here is energy conservation which prevents heating transitions to occur, i.e. those that excite the ion and create a phonon. However, this argument is true only if the driving field is weak thus limiting the cooling rate. In this work we extend earlier work of ours which instead uses the principle of destructive interference to prevent certain undesired transitions. As this interference is independent of the driving rate we can use it to achieve faster cooling. The more off-resonant transitions are excluded the better and our work provides such a scheme.
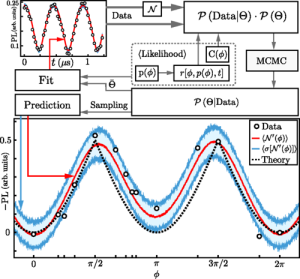
Controllable Non-Markovianity for a Spin Qubit in Diamond – J. F. Haase, P. J. Vetter, T. Unden, A. Smirne, J. Rosskopf, B. Naydenov, A. Stacey, F. Jelezko, M. B. Plenio, and S. F. Huelga, Physical Review Letters, 121, 060401 (2018) | ArXiv
DOI: doi.org/10.1103/PhysRevLett.121.060401
*The figure was reprinted with the permission from the above paper. Copyright 2018 American Physical Society
Usually, protocols in quantum simulation, communication or metrology suffer under the ubiquitous presence of noise. However, recent results show that non-Markovian noise can be less detrimental than Markovian noise. Non-Markovian noise is usually associated with memory effects in the system, that is, the system’s evolution is not only determined by its current state as it would be for Markovian noise.
In this paper, we theoretically and experimentally investigate the nitrogen vacancy (NV) center in diamond as a qubit, who’s evolution can be controlled such that its degree of non-Markovianity can be tuned continuously. The regulator of this dynamics is the NV’s intrinsic nitrogen spin. One of the key advantages that this system provides is the following: The degree of non-Markovianity is solely determined by the initial preparation of the nitrogen spin and hence no intervention during the evolution is required. This is the first demonstration of controllable non-Markovianity in a solid-state system.
To demonstrate the feasibility of the system, we employ Bayesian inference techniques to reduce the required data collection to a minimum. Furthermore, the Bayesian modeling allows the prediction of not yet measured data points and allows the precise characterization of the parameters involved.
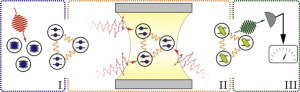
Fundamental limits to frequency estimation: a comprehensive microscopic perspective – J. F. Haase, A. Smirne, J. Kołodyński, R. Demkowicz-Dobrzański, and S. F. Huelga, New Journal of Physics, 20, 053009 (2018) | ArXiv
DOI: doi.org/10.1088/1367-2630/aab67f
*This work is licensed under a Creative Commons Attribution 3.0
When a quantum system is employed as a sensor, the quantity to be estimated is encoded into the quantum state, for example into the phase. However, the outcomes of any measurement we do to determine the state, underly the probabilistic nature of quantum mechanics. In the end, this boils down to the fact that we need to distinguish different probability distributions that are parameterized by the quantity we want to employ the sensor for. Since it is impossible to perfectly determine a probability distribution within a finite number of tries, there is always an error associated with our estimation (one might think for example about a coin, where one aims to estimate the probability to get head or tail).
It was found that using entanglement between different sensors, this error can be smaller than it may be achieved using only classical resources, i.e., measuring more often. However, when the encoding procedure of the parameter is influenced by environmental noise, this quantum advantage quickly vanishes. In this paper, we consider the spin-boson model and examine under which circumstances, an in particular, properties of the induced noise, the quantum advantage is at least partially maintained when we estimate the energy splitting of the spin.
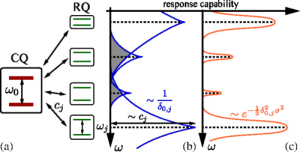
Soft Quantum Control for Highly Selective Interactions among Joint Quantum Systems – J. F. Haase, Z.-Y. Wang, J. Casanova, and M. B. Plenio, Physical Review Letters, 121, 050402 (2018) | ArXiv
DOI: doi.org/10.1103/PhysRevLett.121.050402
*The figure was reprinted with the permission from the above paper. Copyright 2018 American Physical Society
For many applications in the arising field of quantum technologies, we require to couple specific constituents of a cluster of quantum systems, for example when constructing a register of nuclear spins which are controlled by an NV center, or in nanoscale NMR where each spectral component of a probe should be resolved as precise as possible. Thereby, one exploits that transversal interactions between two quantum systems are suppressed when they are out of resonance, that is, their energy splittings are different. To couple two particular systems, one tunes them on resonance, usually via a constant drive or dynamical decoupling. Of course, this resonance does not have to be matched exactly and this “addressability” falls of with the inverse of the detuning of the two systems (the detuning is difference between the energy splittings). This inverse proportionality is not sufficient when further systems are presents, since their energy splitting may only differ slightly and hence the coupling is not very selective.
In this work, we propose to combine the resonance condition with an adiabatic on and off switching of the global coupling Hamiltonian. We find that, when this done according to a Gaussian shape, the addressability falls off exponentially with respect to the detuning. In turn, this allows for a more efficient rotating wave approximation, higher resolution in sensing spectra and high fidelity quantum gates in the vicinity of systems with close energy splittings.
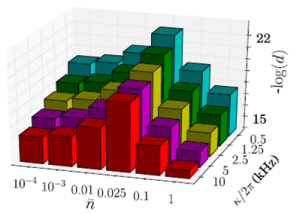
A trapped-ion simulator for spin-boson models with structured environments – A. Lemmer, C. Cormick, D. Tamascelli, T. Schaetz, S. F. Huelga, and M. B. Plenio, New Journal of Physics, 20, 073002 (2018)
DOI: doi.org/10.1088/1367-2630/aac87d
*This work is licensed under a Creative Commons Attribution 3.0
The goal of building a quantum simulator is to construct highly controlled quantum systems which allow us to probe the physics of many-particle systems that are not accessible to simulation on classical computers. Particularly promising in this respect are systems that are composed of both spin and harmonic oscillators as these are particularly hard to simulate numerically. Here we propose a method to simulate the dynamics of spin-boson models with small crystals of trapped ions where the electronic degree of freedom of one ion is used to encode the spin while the collective vibrational degrees of freedom are employed to form an effective harmonic environment. The key idea of our approach is that a single damped mode can be used to provide a harmonic environment with Lorentzian spectral density. More complex spectral functions can be tailored by combining several individually damped modes. The protocol is especially well-suited to simulate spin-boson models with structured environments which is a paradigmatic model for the system-environment interaction with applications for example to exciton dynamics in contact with their vibrational environment in biological systems.
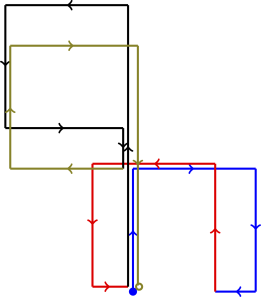
Quantum-optical tests of Planck-scale physics – S. P. Kumar and M. B. Plenio, Physical Review A, 97, 063855 (2018)
DOI: doi.org/10.1103/PhysRevA.97.063855
*The figure was reprinted with the permission from the above paper. Copyright 2018 American Physical Society
Phenomenological models of quantum gravity predict that the usual canonical commutation relations of quantum mechanics have small corrections. Recently, it was proposed to use cavity-optomechanical systems to test for these corrections. In this work, we improve the achievable precision of such devices. More specifically, we develop sophisticated paths in phase space of such optomechanical systems to obtain significantly improved accuracy and precision under contributions from higher-order corrections to the optomechanical Hamiltonian. An accurate estimate of the required number of experimental runs is presented based on a rigorous error analysis that accounts for previously unaccounted mean photon-number uncertainty, which can arise from classical fluctuations or from quantum shot noise in measurement. Furthermore, we propose a method to increase precision by using squeezed states of light. Finally, we demonstrate the robustness of our scheme to experimental imperfection. This analysis improves the prospects of carrying out tests of quantum gravity with near-future optomechanical technology.
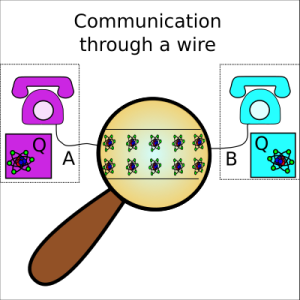
Of Local Operations and Physical Wires – D. Egloff, J. M. Matera, T. Theurer, and M. B. Plenio, Physical Review X, 8, 031005 (2018) | ArXiv
DOI: doi.org/10.1103/PhysRevX.8.031005
This work is licensed under a Creative Commons Attribution 4.0 International License.
One of the oldest questions in quantum mechanics is how quantum states differ from everyday, classical states. While the answer “it depends” is correct, researchers would like to more rigorously understand and quantify this difference. This would help in a number of areas, from understanding the impact of quantum mechanics on biological systems to objective comparisons of different quantum computing platforms. Here, we take a step toward truly understanding what it means for a system to be quantum by unifying different perspectives on the quantum-classical divide.
A clear manifestation of nonclassicality is entanglement. This property can be generated by two spatially separated experimenters, Alice and Bob, if they can send quantum particles to each other but not if they can only communicate classically (e.g., through a phone). Thus, there is no transparent way to make a classical (i.e., stochastic) model for its behavior—entanglement is therefore nonclassical.
However, many systems are neither entangled nor classical. For this reason, more general approaches have been considered to qualify a system as nonclassical. Our theory unifies many of these approaches by modeling the communication between Alice and Bob to be explicitly carried by a physical system, a “wire.” Entanglement between Alice and Bob is then quantified as usual, but the theory is more general, as it allows one to consider the perspective of the wire connecting the two. This theory yields a transparent way to model the wire as exactly classical if it cannot be used to generate entanglement.
Our theory unifies different known nonclassical features and allows us to quantify and compare them. This is important if one wants to understand and meaningfully discuss the value of nonclassicality.
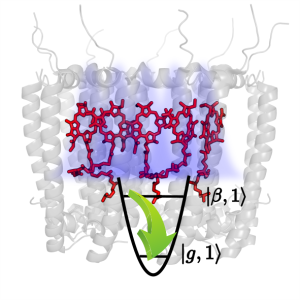
Theory of Excitonic Delocalization for Robust Vibronic Dynamics in LH2 – F. Caycedo-Soler, J. Lim, S. Oviedo-Casado, N. F. van Hulst, S. F. Huelga, and M. B. Plenio, Physical Chemistry Letters, 9, 3446 (2018) | ArXiv
DOI: doi.org/10.1021/acs.jpclett.8b00933
*The figure was reprinted with the permission from the above paper. Copyright 2018 American Chemical Society
Nonlinear spectroscopy has revealed long-lasting oscillations in the optical response of a variety of photosynthetic complexes. Different theoretical models which involve the coherent coupling of electronic (excitonic) or electronic-vibrational (vibronic) degrees of freedom have been put forward to explain these observations. The ensuing debate concerning the relevance of either mechanism may have obscured their complementarity. To illustrate this balance, we quantify how the excitonic delocalization in the LH2 unit of purple bacterium provides adequate conditions for robust coherence in the vibronic dynamics, which helped us to explain the long-lasting oscillations observed in fluorescent traces in LH2, and allowed us to comment on the role of this complementarity in the Fenna-Matthews-Olson complex of green sulphur bacteria.
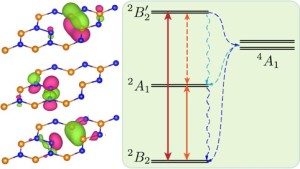
Color Centers in Hexagonal Boron Nitride Monolayers: A Group Theory and Ab Initio Analysis – M. Abdi, J.-P. Chou, A. Gali, and M. B. Plenio, ACS Photonics, 5, 1967 (2018) | ArXiv
DOI: doi.org/10.1021/acsphotonics.7b01442
*The figure was reprinted with the permission from the above paper. Copyright 2018 American Chemical Society
Thanks to the advances in fabrication and control, two-dimensional (2D) materials are under intense investigation nowadays for their potential applications in quantum technology. The recent reports on the observation of single photon emission from few layers of hexagonal boron nitride (h-BN) samples has sparked a considerable interest. The origin of these emitters, nonetheless, is still unknown whilst a better understanding of their origins is required for their control, which cannot be achieved without theoretical investigations. The purpose of our work is to provide an analysis based on symmetry observations in a few of the point defects in h-BN and correlate the experimental observations and our ab-initio calculations to explain the electronic and magnetic properties of the optical emitters via group theory analysis. We have shown that the properties of some of the studied defects allow for their application in quantum information processing.
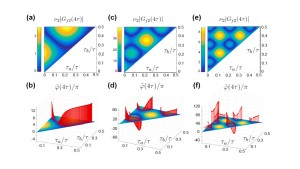
Pulsed dynamical decoupling for fast and robust two-qubit gates on trapped ions – I. Arrazola, J. Casanova, J. Pedernales, Z.-Y. Wang, E. Solano, and M.B. Plenio, Physical Review A, 97, 052312 (2018) | ArXiv
DOI: doi.org/10.1103/PhysRevA.97.052312
*The figure was reprinted with the permission from the above paper. Copyright 2018 American Physical Society
A quantum computer makes use of qubits and engineered interactions among them. Trapped ions offer both, qubits encoded in electronic levels and interactions mediated by their collective oscillation modes. Ideally, after a quantum gate, the qubits should be unentangled from the motional modes such that the quantum algorithm can go ahead. To this end, existing approaches continuously rotate the qubits close to the frequency of a specific mode in order to decouple them from the rest of modes, which oscillate at inconmensurable frequencies. However, this produces gates that are orders of magnitude slower than the oscillation period of the ions. Here, with the advent of microwave control of trapped ions, we propose a pulsed gate scheme that accommodates the dynamics of all the modes, bringing the gate time down to the order of the oscillation period of the ions.
In the pursuit of quantum computers, we require fast and accurate entangling quantum gates, generated with control elements growing in a tractable manner with the system size. We combine these three considerations, introducing a conceptually novel scheme for the generation of quantum gates that opens a new road to the scalable quantum control of trapped ions.
In particular, we numerically optimize the inter-pulse spacing for a sequence of microwave pi-pulses, such that at the end of the sequence the desired phase has been acquired by the qubits, while they are left unentangled from the motional modes. Moreover, the symmetry of our sequence makes it robust against the dominant sources of noise.
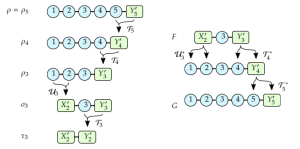
Petz recovery versus matrix reconstruction – M. Holzäpfel, M. Cramer, N. Datta, and M.B. Plenio, Journal of Mathematical Physics, 59, 042201 (2018) | ArXiv
DOI: https://doi.org/10.1063/1.5009658
*The figure was reproduced with the permission of AIP publishing
This work improves and compares methods for the reconstruction of many-particle quantum states. Reconstructing a quantum state of many particles represents a challenge because the Hilbert space dimension grows exponentially with the number of particles. However, under certain conditions on the correlations present in the state in question, the state can be reconstructed from a small number of measurements which grows only linearly with the number of particles. For example, a state with a suitable matrix product state structure may be reconstructed from reduced states of few neighbouring particles in a linear chain. As shown by previous work, the reconstruction may in this case be performed with the so-called Petz recovery map or a suitable, repeated application of a matrix pseudoinverse. We compare the conditions used by the two reconstructions methods. In addition, we improve the two methods by extending them to use long-range measurements in addition to local reduced states, enabling reconstruction of a larger set of states.
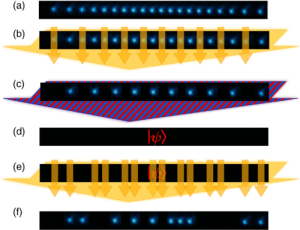
Observation of Entangled States of a Fully Controlled 20-Qubit System – N. Friis, O. Marty, C. Maier, C. Hempel, M. Holzäpfel, P. Jurcevic, M. B. Plenio, M. Huber, C. Roos, R. Blatt, and B. Lanyon, Physical Review X, 8, 021012 (2018) | ArXiv
DOI: https://doi.org/10.1103/PhysRevX.8.021012
This work is licensed under a Creative Commons Attribution 4.0 International License.
At the heart of useful quantum technologies such as simulators or computers lies the ability to create quantum correlations such as entanglement. One challenge is to establish and maintain this fragile property for large numbers of particles. Another challenge is to develop methods to detect entanglement. In this work, we present two methods for entanglement detection and use them to detect entanglement in a system of 20 qubits, the largest fully controllable entangled quantum system to date. In particular, we experimentally study the dynamical evolution of the multipartite entanglement structure of 20 qubits encoded in a quantum simulator built from trapped ions. We track the buildup of entanglement between neighboring qubit pairs and triples, and we even verify genuine quantum correlations for up to 5 neighbors. Most importantly, our evaluation only requires a number of measurement settings proportional to the number of qubits—just 27 out of the more than 3 billion possible measurements. This is in contrast to full state reconstruction, where this number grows exponentially with the system size, which is not viable for scalable entanglement detection.
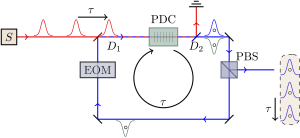
Proposal for Quantum Simulation via All-Optically-Generated Tensor Network States – I. Dhand, M. Engelkemeier, L. Sansoni, S. Barkhofen, C. Silberhorn, and M. B. Plenio, Phys. Rev. Lett. 120, 130501 | ArXiv
DOI: https://doi.org/10.1103/PhysRevLett.120.130501
General quantum states possess a complex entanglement structure that makes their description on a classical computer inefficient in the sense that the computational effort can grow exponentially with the number of subsystems. However, in a wide variety of important states, including the ground and thermal states of local Hamiltonians, the entanglement and correlations are typically more limited as they satisfy area laws. Such states can be approximated well in terms of tensor network (TN) states parametrisation, in which only a polynomial, in the number of subsystems, number of parameters is required to describe the state. Although TNs in one dimension can be efficiently manipulated on a classical computer, the treatment of TN states in higher spatial dimensions remains challenging because the computational effort, while polynomial, grows with a high power in the number of subsystems and bond dimension. Therefore, the experimental generation of TN states and their use for quantum simulation is of considerable interest. Furthermore, the generation of TNs is important as they include important resource states for quantum communication, teleportation and metrology. In our work, we propose an all-optical scheme for the generation of TN states in one and higher dimensions. Our all-optical scheme exploits well established parametric down-conversion methods to build entanglement in the generated state. The scheme also promises robustness against loss and mode mismatch and can be realised with current and near-future optical technology.
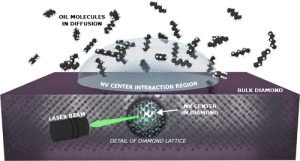
Toward Hyperpolarization of Oil Molecules via Single Nitrogen Vacancy Centers in Diamond – P. Fernández-Acebal, O. Rosolio, J. Scheuer, C. Müller, S. Müller, S. Schmitt, L.P. McGuinness, I. Schwarz, Q. Chen, A. Retzker, B. Naydenov, F. Jelezko, and M.B. Plenio, Nano Letters, 18, 1882 (2018)
DOI: 10.1021/acs.nanolett.7b05175
NV centers in diamond are one of the most promising platforms for the realization of quantum technologies. They possess numerous features that make out of them a powerful tool to access the quantum world. In this work we exploit their strong interaction with surrounding nuclei to build up a hyperpolarization protocol for organic molecules. That is, using the NV center we can efficiently increase the polarization of nuclear spins belonging to different organic molecules. This is of enormous help, for example, for bio-medicals routines such as NMR. Our contribution consists on an extensive theoretical analysis along with numerical simulations that explains and predict polarization transfer between one single NV center and a vast number of nuclei. Our predictions reproduce in a strong agreement the behavior observed experimentally. Together, these results set the route to novel NMR techniques and better medical diagnosis in the near future.
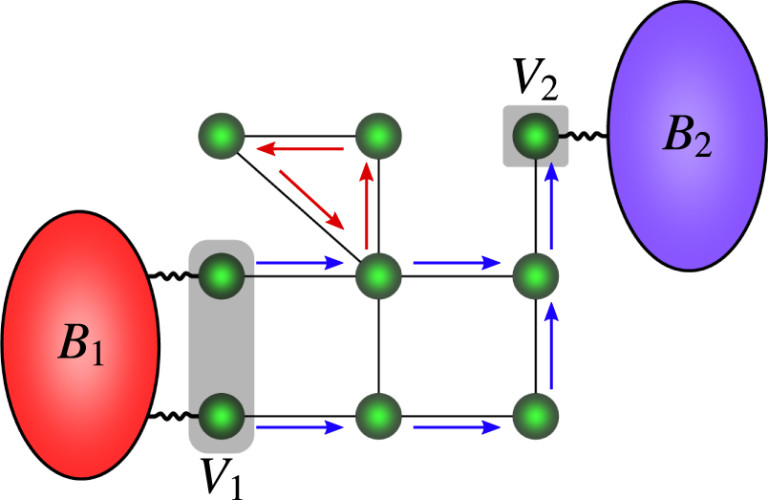
Non-additive dissipation in open quantum networks out of equilibrium – M. T. Mitchison and M. B. Plenio, New Journal of Physics, 20, 033005 (2018) | ArXiv
DOI:https://doi.org/10.1088/1367-2630/aa9f70
Many fundamental and practical problems in physics reduce to studying a network of coupled quantum systems driven out of equilibrium by external noise. This broad paradigm incorporates biological transport networks, critical phenomena in dissipative many-body systems, and next-generation mesoscopic electronic devices. However, the theoretical description of these systems is difficult and typically requires approximations of questionable validity. Here, we shed some light on this problem by studying a simple non-equilibrium network comprising just two coupled subsystems, each of which interacts with an external heat reservoir. This model has some very nice symmetry properties, which help us to solve its dynamics exactly. We show that, when the reservoirs are not in thermal equilibrium with each other, they +ACI-interfere+ACI with each other in the sense that their effect on the network cannot be described as a sum of independent contributions. This interference is absent from standard descriptions based on master equations and can even lead to highly non-Markovian dynamics. Remarkably, the effect persists even when the reservoirs are spectrally unstructured and weakly coupled to the open system. Our research contributes to a body of recent work highlighting the subtle interplay between multiple noise sources in dissipative quantum networks.
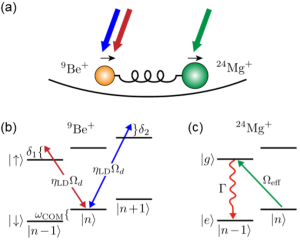
Dissipative phase transition in the open quantum Rabi model – M.-J. Hwang, P. Rabl, and M. B. Plenio, Physical Review A, 97, 013825 (2018) | ArXiv
DOI:https://doi.org/10.1103/PhysRevA.97.013825
In open quantum systems, a delicate interplay between coherent interactions and dissipations can bring a system into a non-equilibrium steady state that undergoes an abrupt and non-analytical change, marked by a so-called dissipative phase transition (DPT). In this work, we provide arguably the simplest model system, consisting of a damped harmonic oscillator and a qubit described by the so-called open quantum Rabi model, that exhibits a DPT, by extending the notion of finite-component system phase transitions to an open quantum system. We propose a trapped-ion experiment where the predicted DPT can be realised. Our proposal allows one to control the strength of dissipation and even turn on and off the dissipation so that one can switch from realizing a quantum phase transition to dissipative phase transition; this unique feature of our proposal opens a door for exploring the dynamics of dissipative phase transition in a highly controlled fashion.
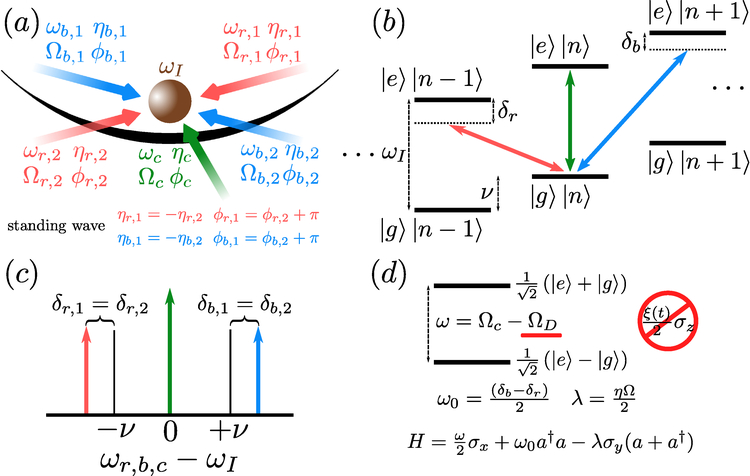
Magnetic field fluctuations analysis for the ion trap implementation of the quantum Rabi model in the deep strong coupling regime – R. Puebla, J. Casanova, and M. B. Plenio,
J. Mod. Opt. (Special Issue: Quantum optics, cooling and collisions of ions and atoms), 603-611 (2017) | ArXiv
DOI: https://doi.org/10.1080/09500340.2017.1404651
Light-matter interactions are of paramount importance for several applications, such as in quantum-based technologies. In particular, triggered by an impressive experimental progress and theoretical works, which have pointed out the suitability of these systems for quantum information processing, sensing and simulation, the study of light-matter systems has experienced a renewed interest. In this regard, the quantum Rabi model (QRM) appears as the simplest, yet fundamental, system describing the interaction between a spin (matter) and a bosonic mode (light).
In this work we have extended previous works [New J. Phys. 18, 113039 (2016) and Phys. Rev. A, 95, 063844 (2017)] where a noise-resilient trapped-ion realization of QRMs based on continuous dynamical decoupling techniques was proposed. While trapped ions are prone to different imperfections, magnetic-field fluctuations are considered as the main source of noise, and hence its suppression is highly desired to properly retrieve QRM’s physics. Nevertheless, depending on the desired and targeted parameter regime of the QRM, one may resort to different dynamical decoupling schemes. As an example, we show here how to attain a QRM in the striking deep-strong coupling regime while suppressing the effects of the magnetic-field fluctuations – an inaccessible regime relying on the previously proposed schemes.
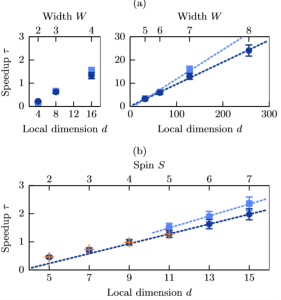
Probabilistic low-rank factorization accelerates tensor network simulations of critical quantum many-body ground states – L. Kohn, F. Tschirsich, M. Keck, M. B. Plenio, D. Tamascelli, and S. Montangero, Physical Review E, 97, 013301 (2018) | ArXiv
DOI: https://doi.org/10.1103/PhysRevE.97.013301
Tensor Networks(TN) methods are indispensable tools in simulating quantum and classical many-body problems by providing an efficient parametrization of the wave function in the many-body phase space. In order to find such efficient parametrization, truncated Singular Value Decomposition (SVD) is widely used to compress states into their respective TN manifold. The SVD lies therefore at the heart of many TN methods, but also represents the most time-consuming part of a wide class of TN algorithms.
In this work we demonstrate that a randomized version of SVD (RSVD), which was proven to reduce the complexity of the Time-Evolving-Block-Decimation TN algorithm [D. Tamascelli, R. Rosenbach, and M. B. Plenio, Physical Review E 91, 063306 (2015)], can be applied to a relevant class of many-body systems, namely systems undergoing a quantum phase transition. Such regime is much more challenging regime since long-range correlations are building up and risk to compromise the effectiveness of the RSVD compression. We provide evidence that RSVD delivers the same accuracy as standard SVD routines with speed-up that can go up to 24 times. We show that the accuracy of the results is not influenced by the speedups and discuss the impact of techniques typical for TN studies.
Contact
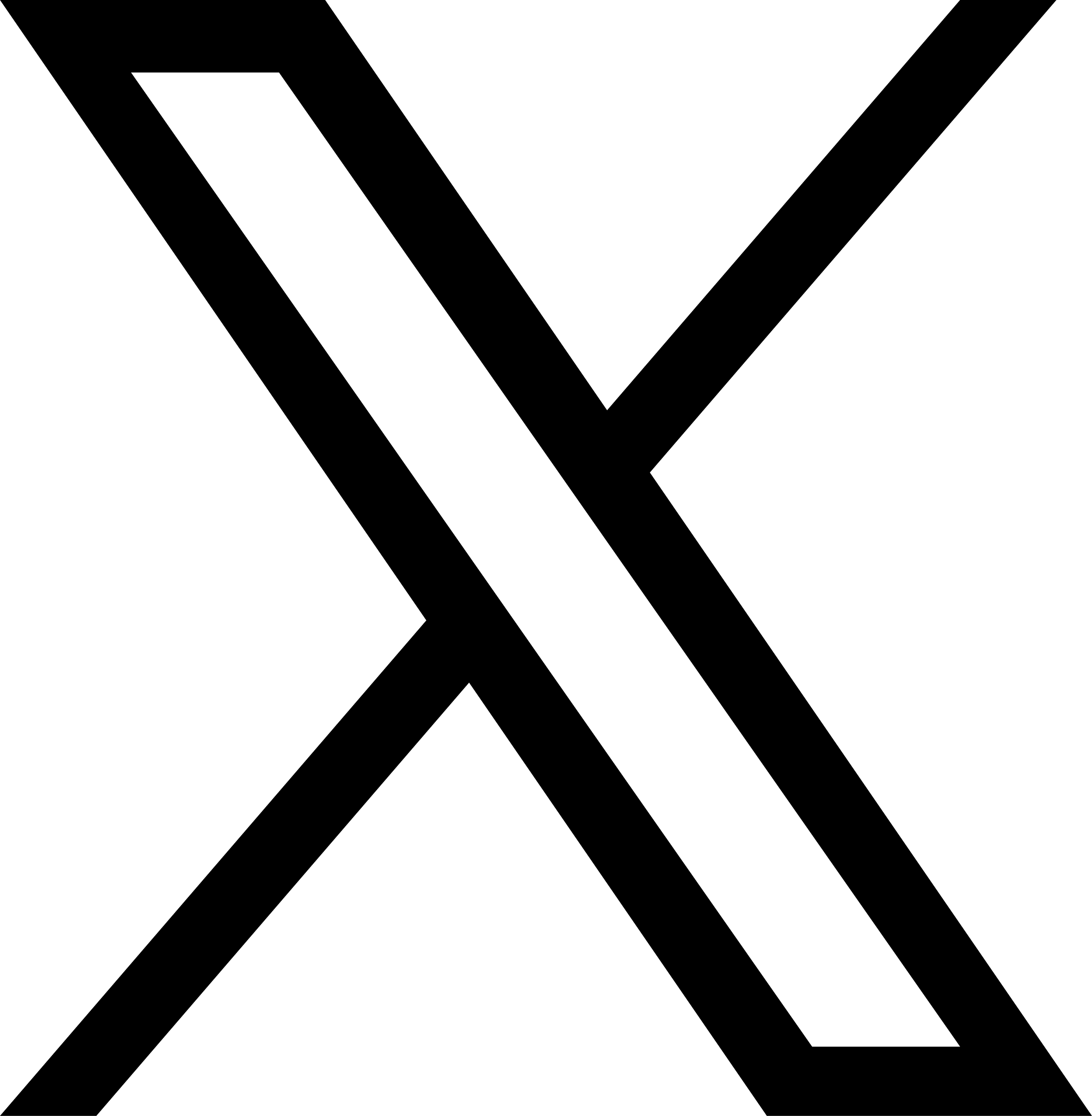

Ulm University
Institute of Theoretical Physics
Albert-Einstein-Allee 11
D - 89081 Ulm
Germany
Tel: +49 731 50 22911
Fax: +49 731 50 22924
Office: Building M26, room 4117
Click here if you are interested in joining the group.
Most Recent Papers
Efficient Information Retrieval for Sensing via Continuous Measurement, Phys. Rev. X 13, 031012, arXiv:2209.08777
Active hyperpolarization of the nuclear spin lattice: Application to hexagonal boron nitride color centers, Phys. Rev. B 107, 214307, arXiv:2010.03334
Driving force and nonequilibrium vibronic dynamics in charge separation of strongly bound electron–hole pairs, Commun Phys 6, 65 (2023), arXiv:2205.06623
Asymptotic State Transformations of Continuous Variable Resources, Commun. Math. Phys. 398, 291–351 (2023), arXiv:2010.00044
Spin-Dependent Momentum Conservation of Electron-Phonon Scattering in Chirality-Induced Spin Selectivity, J. Phys. Chem. Lett. 2023, 14, XXX, 340–346, arXiv:2209.05323