D. Geiger, M. Mundszinger, U. Kaiser
Lithium and post-lithium battery materials
Lithium is an ideal material for batteries because of the high potential and low weight. As cathode for lithium ion batteries intercalation compounds are used to achieve higher security and reduce degradation occurring with metallic lithium. The intercalation battery compounds allow that lithium ions compensate for the charge through the electrolyte when electrons power the electrical consumer. On the anode side, materials like graphite are used for intercalation of lithium.
The currently used lithium batteries contain materials including rare earths which suffer from high and steadily rising prices. For example, materials like nickel and manganese are tested to replace cobalt. To circumvent similar problems with lithium, post lithium battery materials are investigated. Possibilities to apply lithium-free materials, where, e.g., sodium takes over the role of lithium, are currently explored.
A large number of different materials both on anode and cathode side are analysed using electron microscopic methods:
- By means of scanning electron microscopy (SEM), we study the porous and/or granular structures of different anode materials. We obtain the three-dimensional structure of the materials by the focussed ion beam (FIB) tomography technique.
- With high-resolution transmission electron microscopy (HRTEM), the atomic structure of the battery materials can be studied. In order to identify their crystalline structures, we compare the HRTEM images to image simulations.
- Electron energy-loss spectroscopy (EELS) offers the possibility to study the chemical composition and properties.
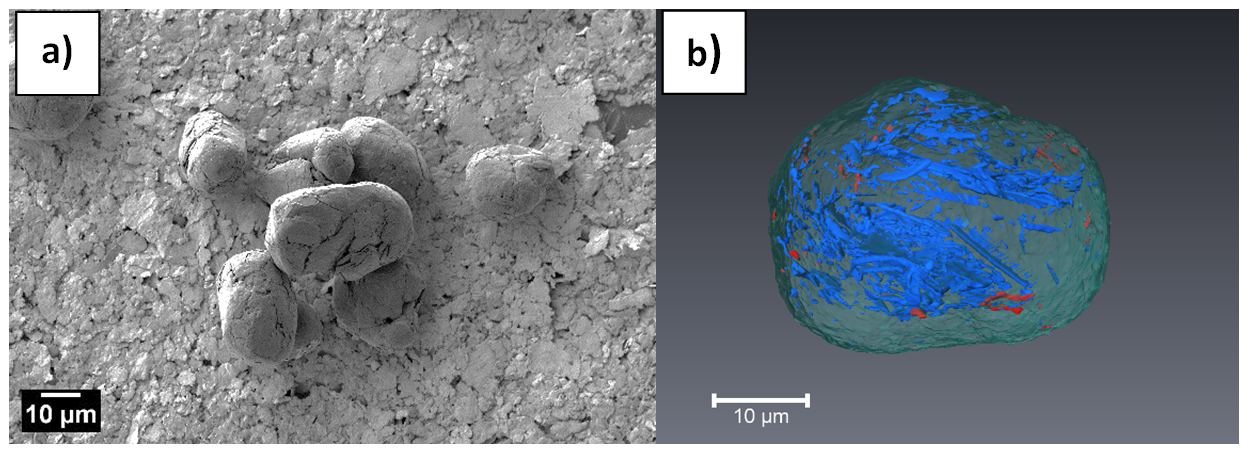
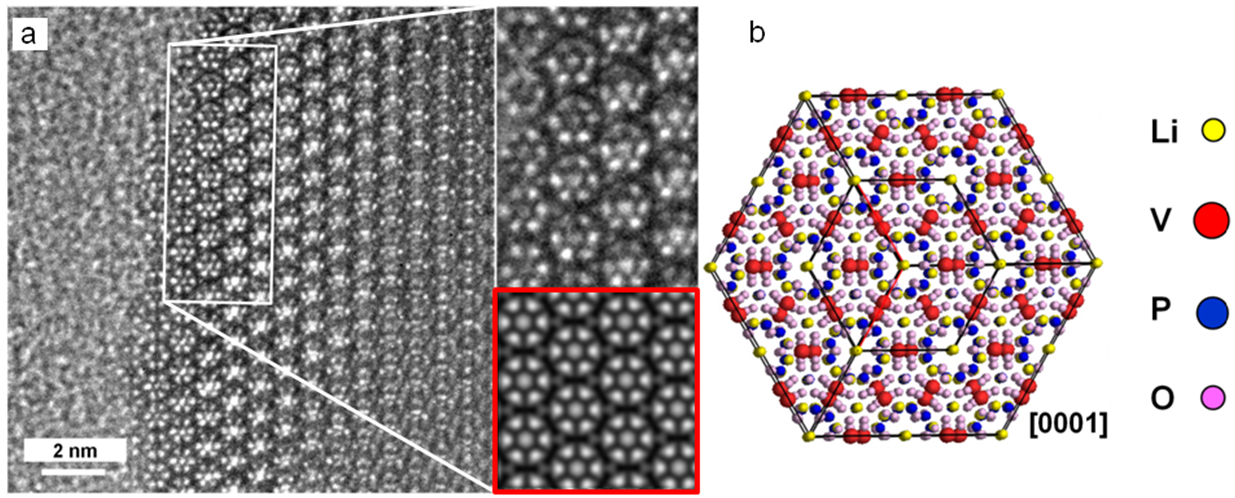
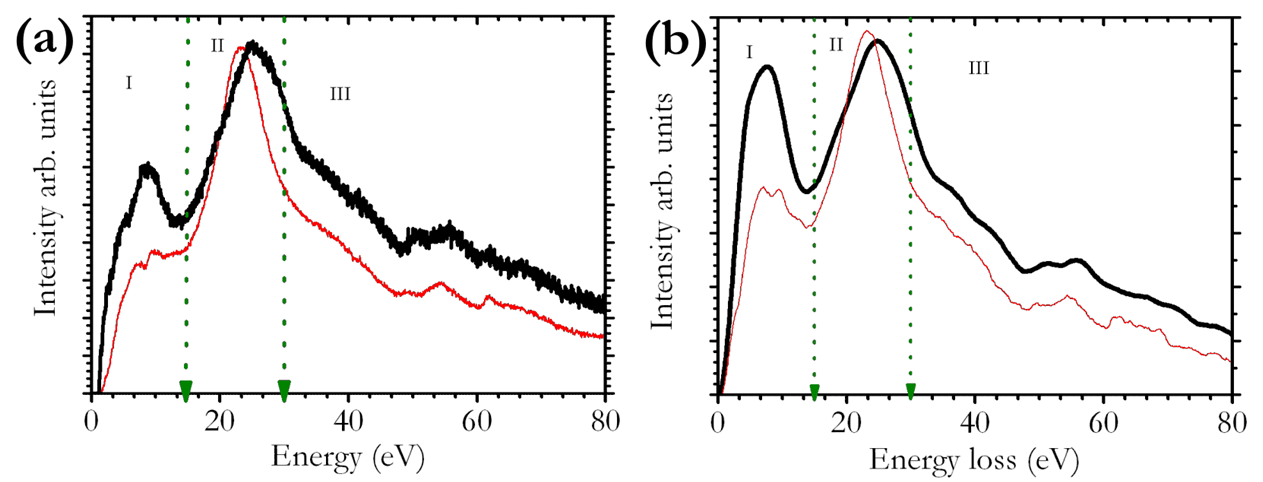
[1] P. Axmann, G. Gabrielli, M. Wohlfahrt-Mehrens, Tailoring high-voltage and high-performance LiNi0.5Mn1.5O4 cathode material for high energy lithium-ion batteries, In Journal of Power Sources, Volume 301, 2016, Pages 151-159, ISSN 0378-7753, doi.org/10.1016/j.jpowsour.2015.10.010.
[2] Manuel Mundszinger, Sarvenaz Farsi, Manfred Rapp, Ute Golla-Schindler, Ute Kaiser, Mario Wachtler, Morphology and texture of spheroidized natural and synthetic graphites, In Carbon, Volume 111, 2017, Pages 764-773, ISSN 0008-6223, doi.org/10.1016/j.carbon.2016.10.060.
[3] P. Balasubramanian, M. Mancini, H. Geßwein, D. Geiger, P. Axmann, U. Kasier and M. Wohlfahrt-Mehrens, Kinetics and structural investigation on layered Li9V3(P2O7)3(PO4)2as cathode material for Li-ion batteries (submitted)
[4] H. Zhang, I. Hasa, D. Buchholz, B. Qin, D. Geiger, S. Jeong, U. Kaiser and S. Passerini, Exploring the Ni redox activity in polyanionic compounds as conceivable high potential cathodes for Na rechargeable batteries, NPG Asia Materials (2017) 9.
[5] B. Qin, H. Zhang, T. Diemant, D Geiger, R. Raccichini, R. J. Behm, U. Kaiser, A. Varzi, S. Passerini, Ultrafast Ionic Liquid-Assisted Microwave Synthesis of SnO Microflowers and Their Superior Sodium-Ion Storage Performance, ACS Appl. Mater. Interfaces 2017, 9.
[6] M. K. Kinyanjui, P. Axmann, M. Mancini, G. Gabrielli, M. Wohlfahrt-Mehrens, F. Boucher, and U. Kaiser, Spectroscopic signatures of Mn valency changes in both valence and core loss spectra in Li1+xNi0.5Mn1.5O4 (x = 0, 1) (submitted)
Dr. Wohlfahrt-Mehrens, ZSW |
Prof. Passerini, HIU |
BMBF in the frame of the LiEcoSafe and Rondo projects